A Sputnik for the 21st Century
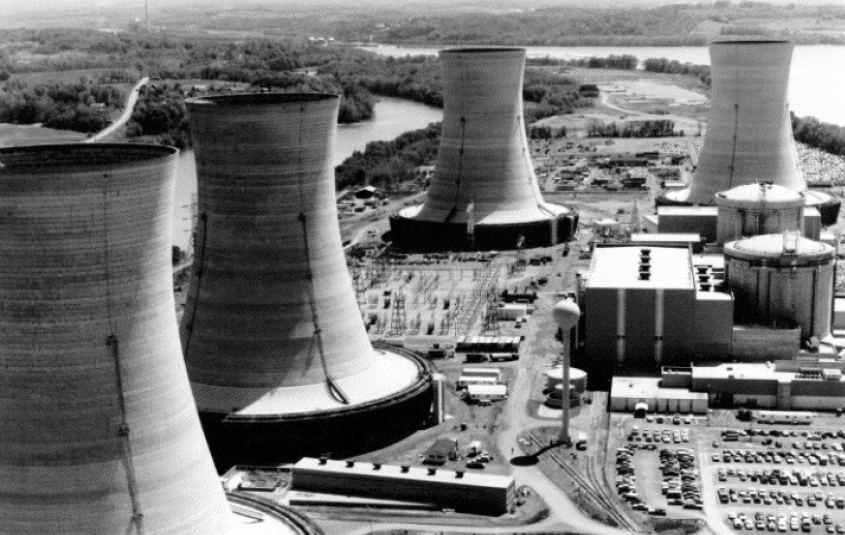
BY NELSON GOMES
On March 18th, the Chinese government announced its intentions to accelerate production of the world’s first commercially viable liquid fluoride reactor. The project, spearheaded by the Chinese Academy of Sciences, was originally allocated a budget of $350 million and scheduled for completion by 2039, but it has since seen its funding grow and its deadline shortened to 2024. With 140 full-time Ph.D. students and a staff of roughly 750 people devoted to the project, let’s hope they’re successful. But more importantly, let’s hope we’ll follow their lead.
It’s not surprising that China has decided to follow a path towards energy independence with nuclear power. Its current base sources of energy, coal and oil, places it in a precarious position in terms of both national security and public health. Russia, from which China buys most of its oil, has become increasingly antagonistic towards the international community, putting China in an uncomfortable position as it tries to develop stronger economic and intellectual ties to Western nations. In addition, coal, despite being one of the most abundant resources on the Chinese mainland, produces dastardly amounts of CO2 , contributing to climate change and resulting in dangerous levels of smog in major cities. “In the past the government was interested in nuclear power because of the energy shortage. Now they are more interested because of smog,” said Professor Li Zhong, who works with the project. Moreover, with twenty eight “regular” nuclear plants in production, China is boldly letting go of the stigma that has plagued nuclear power for decades and is on pace to challenge the United States for the title of largest producer of nuclear energy.
China is not alone in the problems it faces. As the top consumer of energy in the world, the United States’ economic, political, and military hegemony is intrinsically linked to its energy production. While oil will still be a significant geopolitical concern for us in the foreseeable future, we are well within our capacity to dictate our domestic electrical production. According to the Electricity Information Agency (EIA), approximately 67 percent of our electricity comes from coal or natural gas, 20 percent from nuclear reactors, and only 11 percent from all renewable sources combined, of which hydropower alone makes up half. Since the United States has the largest coal reserves in the world and a significant amount of accessible gas reserves, it is tempting to shelf our energy concerns and to continue to rely on our fossil fuels. However, as the Chinese example shows, increasing demand for energy will continue to blow millions of tons of CO2 into our atmosphere, creating debilitating, not to mention expensive, health and climate problems. Moreover, renewable energy sources are incapable of supplying the base power levels demanded from residential and industrial sectors.
Often these renewables cost more per kilowatt-hour (kWh) than their fossil fuel and nuclear counterparts do. According to the DOE’s Annual Energy Outlook 2013, wind and solar sources, subject to changing seasons and hours, only deliver power intermittently and can cost anywhere from $.09 to $.22/kWh for wind and $.14/kWh for solar; nuclear costs a consistent $.11/kWh, which is potentially cheaper than coal ($.10-.14/kWh) and natural gas ($.07-.13/kWh).
The United States certainly stands to gain from nuclear energy. While the intellectual property for the liquid fluoride thorium reactor (LFTR or “lifter”) will be available to the international community, the United States could and should do more to expand its nuclear output and develop new reactor models and technologies. Today, there are just over 100 nuclear reactors licensed and operating in the United States: roughly 70 percent are Pressure-Water Reactors (PWRs) and 30 percent are Boiling-Water Reactors (BWRs). Both models belong to a general class of reactor called the Light-Water Reactor (LWR), which has been the industry norm both here and abroad since its inception. Many of the drawbacks of nuclear power that environmentalists and pragmatists cite are mostly limitations of only the LWR design. The risk of meltdowns, Fukushima-type explosions, unmanageable waste, and increased nuclear proliferation, are all products of a design standard chosen because of its practicality for military use and bomb creation.
However, unlike LWRs, Molten Salt Reactors (MSRs), such as the proposed LFTR reactor, do away with the necessity for water coolants, pressurized operation, long-lived waste, and plutonium byproducts useful for atomic bombs. Safety precautions, such as passively cooled drainage tanks, are inherent in their design, making meltdowns nearly impossible. Additionally, the waste produced from the thorium-uranium cycle would only need to be in storage for about 300 years rather than the often-touted 26,000 years and only a fraction of the LWR waste would, in absolute terms, be produced. Moreover, MSRs use salt solution coolants with high boiling points meaning that they need not operate at high pressure like Fukushima-type reactors, taking away their explosive potential. Lastly, they produce far less byproducts suitable for bomb production. LFTRs also have the added value of their main fuel, thorium, being four times more abundant than uranium and even more energy dense. Therefore, in almost all respects, these reactors are safer and vastly more efficient than their LWR counterparts.
Despite all the advantages and promises that LFTR and other MSR reactors hold, there are other problems intrinsic to the energy industry that can result in devastating consequences due to the nature of radioactivity. France is often touted as being the world leader in nuclear energy. Although they do not produce quite as much electricity, in absolute terms, as the U.S., nearly 80 percent of their electricity comes from nuclear power, and they suffer few if any serious incidents or leaks. Because they have a nationalized nuclear industry, controls and standards are tightly uniform and regulated. The U.S., by contrast, allows the private sector to construct and manage nuclear plants with limited governmental oversight. Hence the main and most pressing issue for the U.S. with regards to nuclear energy: the intersection of money and politics.
Nuclear plants can cost upwards of $1 billion dollars to construct, and as such, are massive enterprises, requiring large federal subsidies to supplement private funding. Even so, profits can take years, sometimes decades to pay back the initial investment. As a result, many of the regulation-skipping, moneysaving tactics that are commonplace in natural gas fracking and oil drilling occur at nuclear power plants as well. Three Mile Island and Indian Point are poignant examples of how weak regulations can lead to potentially devastating leaks or accidents. Despite the fact that proper commitment to regulations ensures radioactivity containment and that the unavoidable exposure is well below any harmful levels (sometimes at orders of magnitude less than what is absorbed by natural background radiation), accidents create stigmas and fears of the development and expansion of the industry due to the uncomfortable sense of imminent exposure. Thus, the U.S. still has a long way to go in fostering the culture of responsibility and obligation that can overcome the pull of compromising profits. Of course, a tougher and better funded Nuclear Regulatory Commission is a welcome prospect, as well.
The 21st century has proven itself to be an extraordinarily expansive and ever-more demanding era for the human race. Computational capacities grow exponentially, population growth rates are still unsustainably high, and our demand for energy is higher than it’s ever been before and is expected to continue rising indefinitely. If we are to keep up with the demands of a globalized society we must find a way to power all of our electronic necessities. Nuclear is the clear answer, but we can only develop and refine it when we remove the stigma and have an honest discussion on how, not whether, we exploit the atom.